How Old is That Rock?
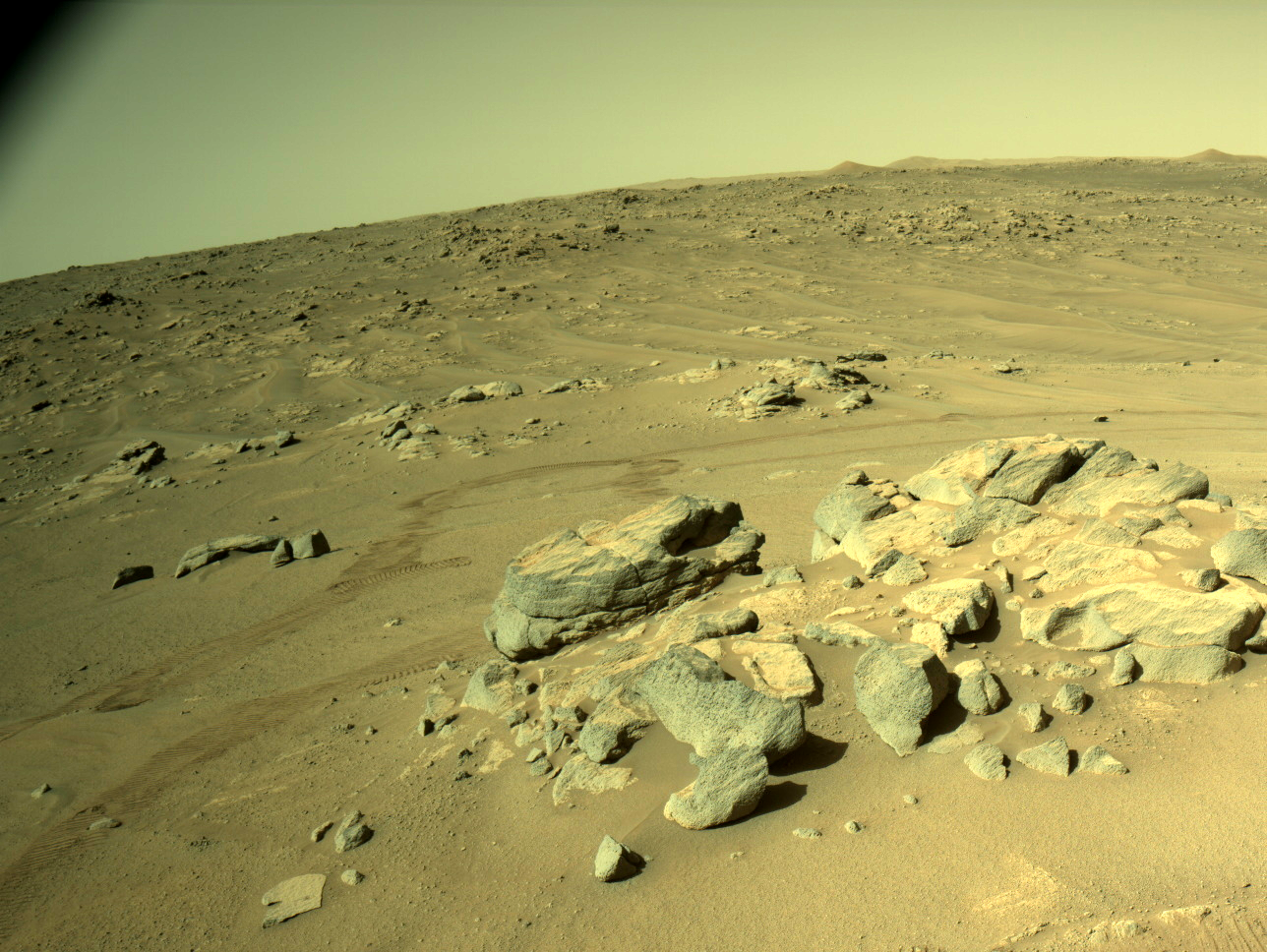
On my first geological field trip as a new geology major at Caltech, I was amazed as we drove out into the Mojave Desert on Interstate 15 when my professor, who was driving, would point through the window at a distant rock outcropping and say: “There’s a Miocene (five to 20 million years of age) sandstone.” How could he determine the age of a rock while driving (fast) along the freeway? Later, I found out that others had done the work and published the results in maps and reports. A good series for armchair geologists is the Roadside Geology books which give detailed road logs along popular routes like I-15, Highway 395 up Owens Valley, or the 101.
One of the key things that geologists do is to try to reconstruct, like a detective, what happened and when it happened. We’re aided by the fact that most geological phenomena produce layers of rock, starting with the oldest at the bottom and getting younger as we go up. We can sometimes also see how one geological unit overlaps another and get an idea which is younger. Those are ways to determine the relative ages of geological units. Other ways include looking at how well developed the soil is on a unit, whether the rocks on a glacial moraine are fresh looking or heavily weathered, and even if there’s a lot of lichen growing on the exposed rocks. It’s better than nothing, but what we really want is the actual age of the rocks.
Early on, as geology was developing as a science, people noticed that certain layers of rock cropped out over large areas. Were these isolated outcrops all related? At the same time, paleontologists were finding fossils in various rock layers that seemed to tell a story of slow evolution of organisms. It seemed that certain assemblages of fossils defined eras of Earth’s history and so the various names of those eras and epochs were invented to systematize that knowledge. Thus, the Cambrian Period (now known to be about 485-540 million years ago) is marked by crab-like trilobites and the Miocene Epoch is characterized by land animals like today’s species.
Using the growing amount of fossil knowledge to correlate widely spaced rock outcrops allowed geologists to make maps showing how far-apart outcrops were related both in space and time. Maps were made that allowed a better understanding of how a region evolved over time: layers of siltstone and clays represented an ocean with the appropriate fossils, but the younger layers maybe were sandstones laid down by rivers after the continents shifted and the seas departed.
Fossils were all well and good, but it was desired to have a numerical age for the rock layers being mapped. How much time was represented by those siltstones? Finally, at the turn of the 20th century, radioactive decay was discovered, leading Ernest Rutherford to propose in 1905 that the age of the Earth could be determined.
Most people have heard of carbon dating, or the use of carbon isotopes to date a material belongs to a family of dating techniques called radiometric dating. What we’re doing is counting how much of a radioactive isotope of an element contained in the sample has decayed into its so-called “daughter” isotopes or elements. It turns out there’s a lot of radioactive isotopes out there, not just uranium and such. The key to dating is to pick an element and its radioactive isotope that decays at a rate that matches your age range of interest.
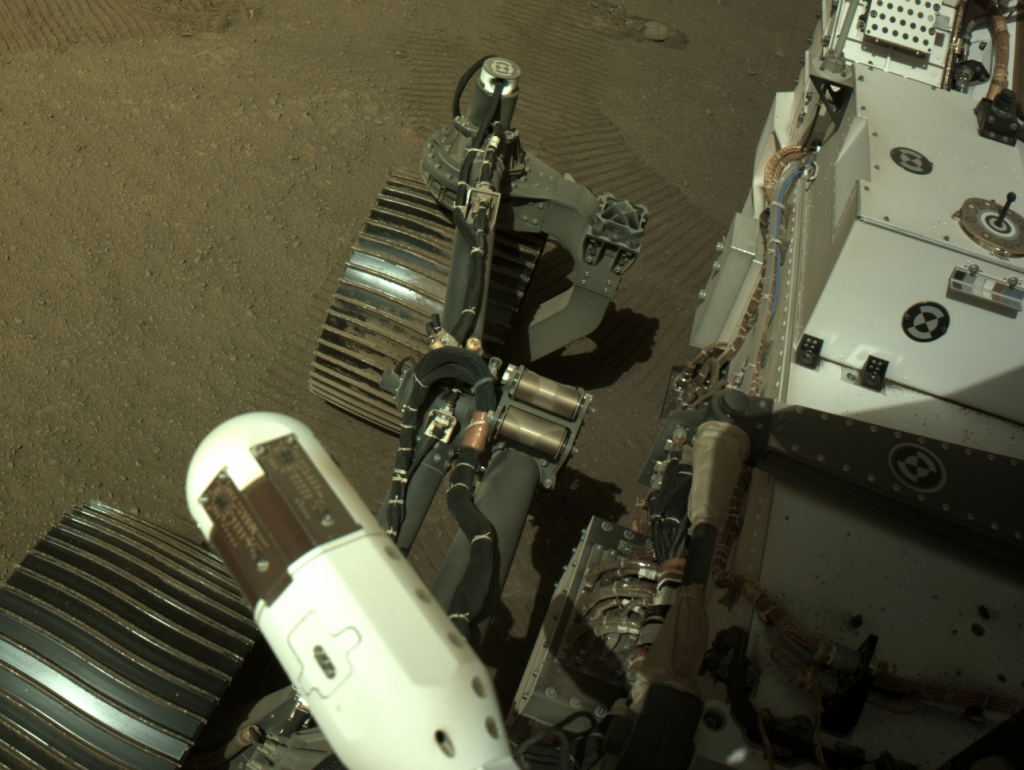
The decay rate is specified as the ‘half-life’ which is just the amount of time for half a sample to decay. For example, the radioactive isotope of carbon is Carbon-14 which means it has two extra neutrons than regular Carbon-12. Carbon-14’s half-life is 5,700 years, which means if you seal off 1 gram of Carbon-14 and come back 5,700 years later, you’ll only find half a gram left, the rest changing to Nitrogen. Since we know the original ratio of Carbon-14 to Carbon-12 in the atmosphere and therefore the sample, we can figure out how much Carbon-14 has decayed by checking the current ratio, yielding the age since the carbon was sealed off from the atmosphere (i.e., the plant died).
Other radioactive isotopes are used for other age ranges. Uranium-238’s half-life is about 4.5 billion years while U-235’s is about 700 million years. Thorium is about 14 billion years, while Potassium-40’s is only about 1.3 million years. So, the geologist has a wide range of isotopes from which to choose.
Another consideration for radiometric dating is that there must be enough of the isotope to be measured. With the capabilities of modern analytical labs, we can measure the ratios to astonishing accuracies; we can now push Carbon dating up to about 60,000 years, or 10 times its half-life. But that’s assuming there’s a decent amount of carbon to start with.
Geologists often spend an inordinate amount of time searching for charcoal or other organic material buried with rocks of interest, but in arid regions, they often come up short. But about 20 years ago, some geologists got so desperate they came up with a brilliant idea: how about turning the tables and look for a build-up of radioactive isotopes in rocks that had been exposed at the surface of the Earth for a long time? The idea was cosmic rays, which are constantly bombarding the planet, can convert elements within rock minerals from ordinary to radioactive isotopes in large enough amounts to be measured.
Sure enough, amounts of radioactive Beryllium, Aluminum, Helium, Chlorine and others have been detected and measured, leading to a new area of study called rock exposure dating, or more technically, cosmogenic radionuclide dating. It’s revolutionized our understanding of landscapes — how long they’ve existed, and how quickly they change and erode. Unfortunately, you typically need a lot of rocks to get a good enough exposure age. On a recent expedition to western China, my team and I had to collect many pounds of rocks from the surfaces of alluvial fans we wanted to date. Then we had to go to the local bazaar in Kashgar and have boxes made to ship them back to the U.S. for analysis. But we got some good numbers that we could relate to past ice ages and earthquake fault slip rates in the area.
Another recent innovation that has allowed us to estimate how long a soil deposit has been sitting undisturbed, is an even more arcane technique called optically stimulated luminance (OSL) dating. The idea here is like rock exposure dating, except the source of the signal is radioactive elements buried with the soil. Over a long period of burial, the radioactive decay causes defects in the mineral crystals surrounding the radioactive particle. The defects build up, but if exposed to sunlight, the sun’s rays erase the defects, resetting the clock. Thus, the soil samples must be collected at night and sealed until they’re brought to the lab and exposed to a special lamp while a photometer records the small amount of light given off by the sample as the crystal defects are healed. It works and has led to insights into how soils and landforms develop and persist.
The isotopic ratios we use for dating are measured with a mass spectrometer, which takes a vaporized sample, ionizes it, and then fires the ionized gas through a strong magnetic field which bends the ionized beam proportionally to the isotopic mass. All that sample preparation, ionization, and acceleration are difficult to accomplish well in a small package which is why we still can’t date rocks accurately on other planets. A trial was conducted with a mass spectrometer designed for another purpose on the Mars rover Curiosity, but the sensitivity was a little low for accurate rock dating. So, it’s still necessary to bring samples back to Earth for analysis in our big machines. Lunar samples brought back by the Apollo astronauts were successfully dated and the Mars rover Perseverance is currently collecting samples to cache them for a future Mars Sample Return mission which will bring them back.
So next time you’re planning a road trip, consult a roadside Geology book so you can wow your passengers by calling out the ages of the rock formations you pass.